I have a repaired sweater with drawstrings and cord locks on the cuffs, which combines the comfort of long, loose sleeves with the ability to snug them up at my elbows for work. I thought it would be a neat exercise in engineering and art to design and make my own cord locks.
For the design, I looked at my personal cord locks, at cord locks online and their reviews. From the reviews I learned to be cautious about designing with too weak a spring. Aesthetically, I made a few CAD sketches until I found something I liked in a similar style as the belt buckle I made. A big concern of mine was that I would enjoy the appearance regardless of the position, and that all features would look intentional. For example, the alignment of the mobile part is maintained relative to the stationary part by having an elliptical track. One of my designs had a sort of terraced top made from the two parts so regardless of whether it was bottomed out or not, it would never transition between a flat top and a terraced top. For the spring, I found one on mcmaster that had the right range and enough compressive force.
This is machined from delrin, with a stainless steel spring and a piano wire pin.
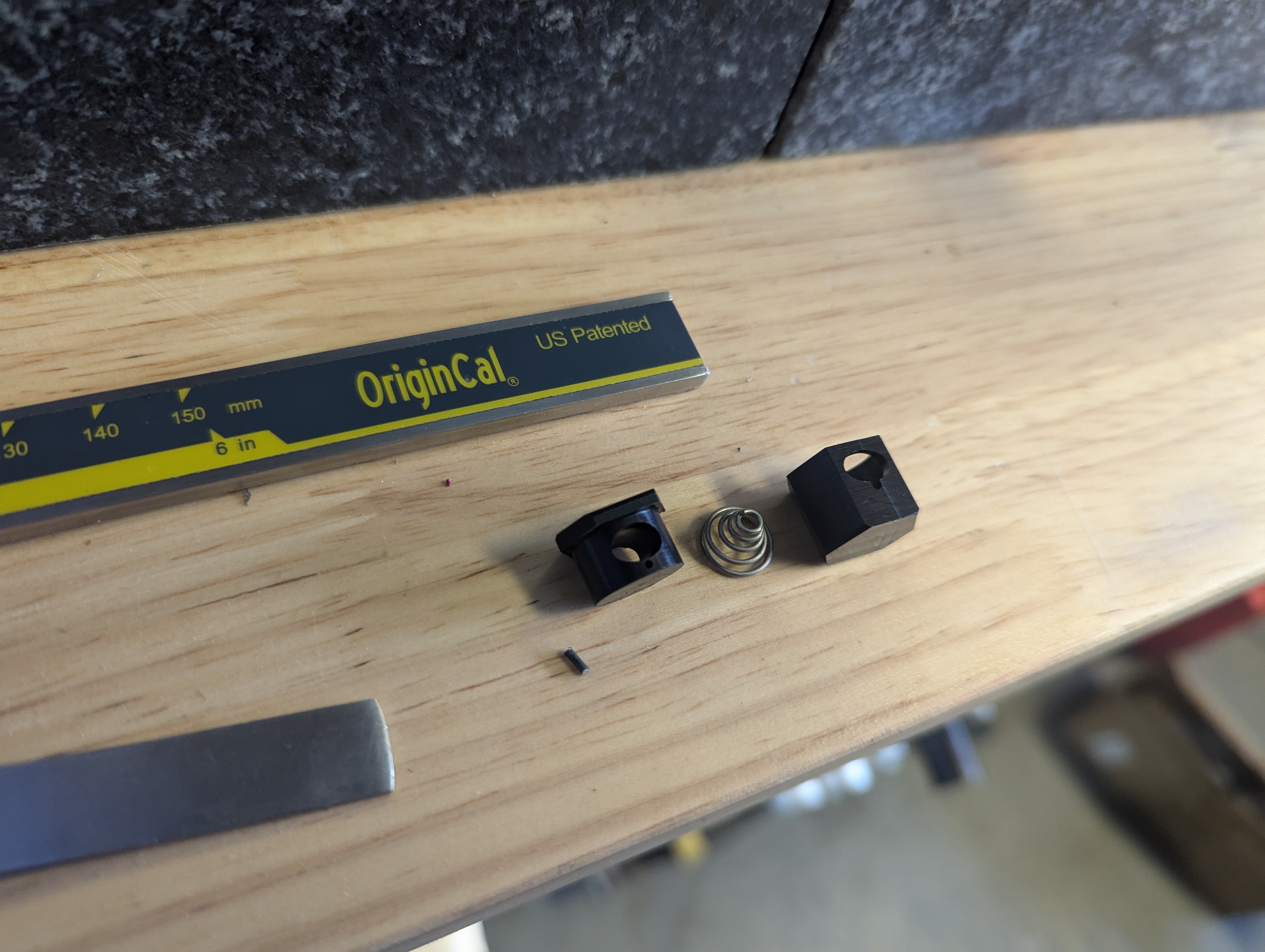
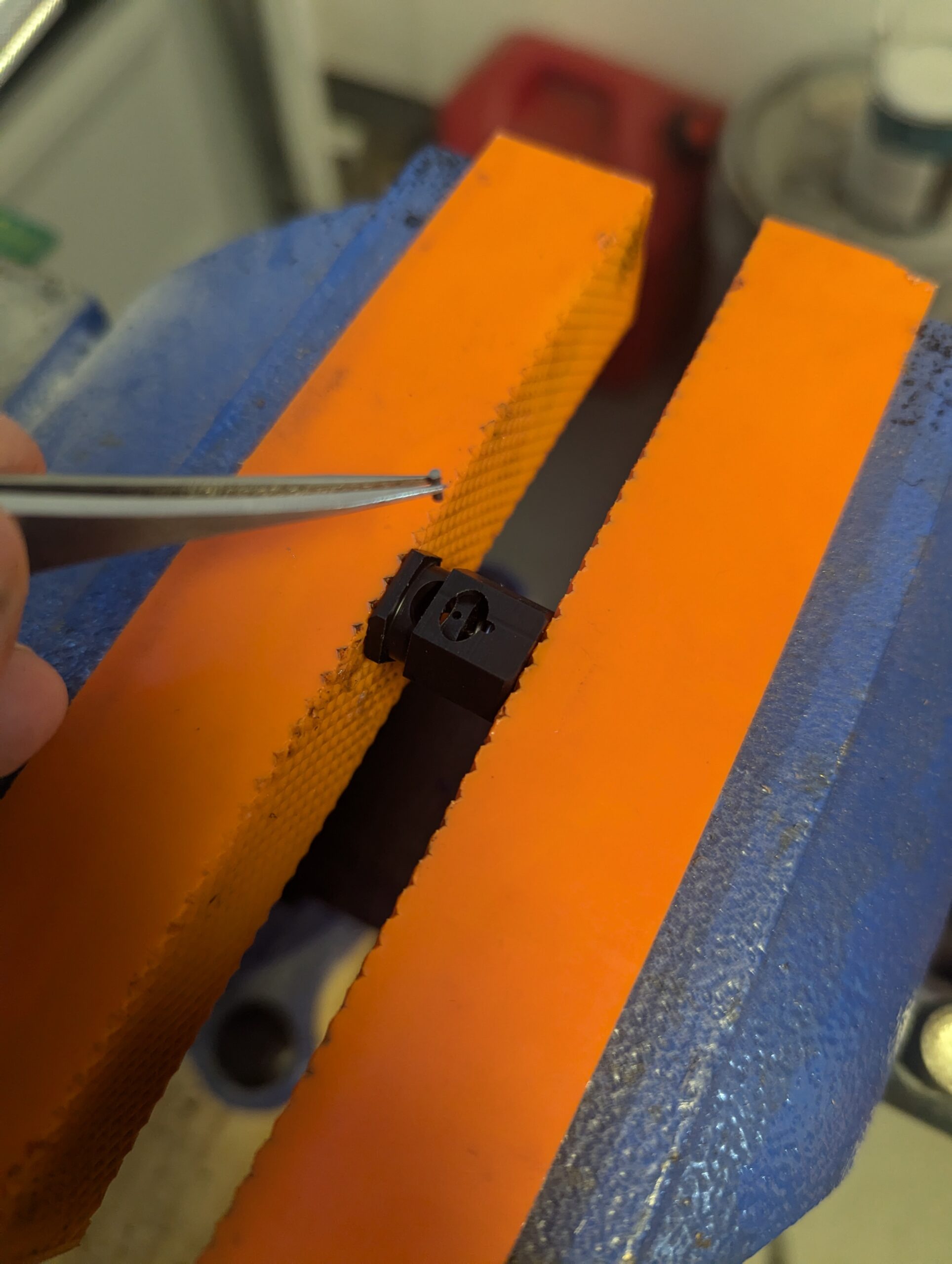
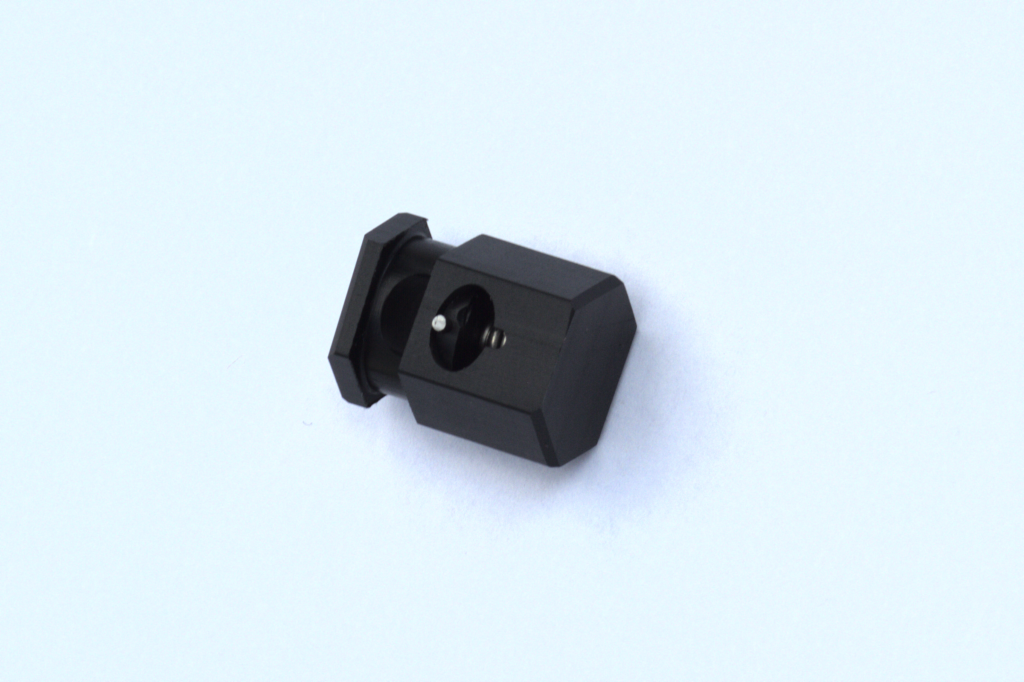
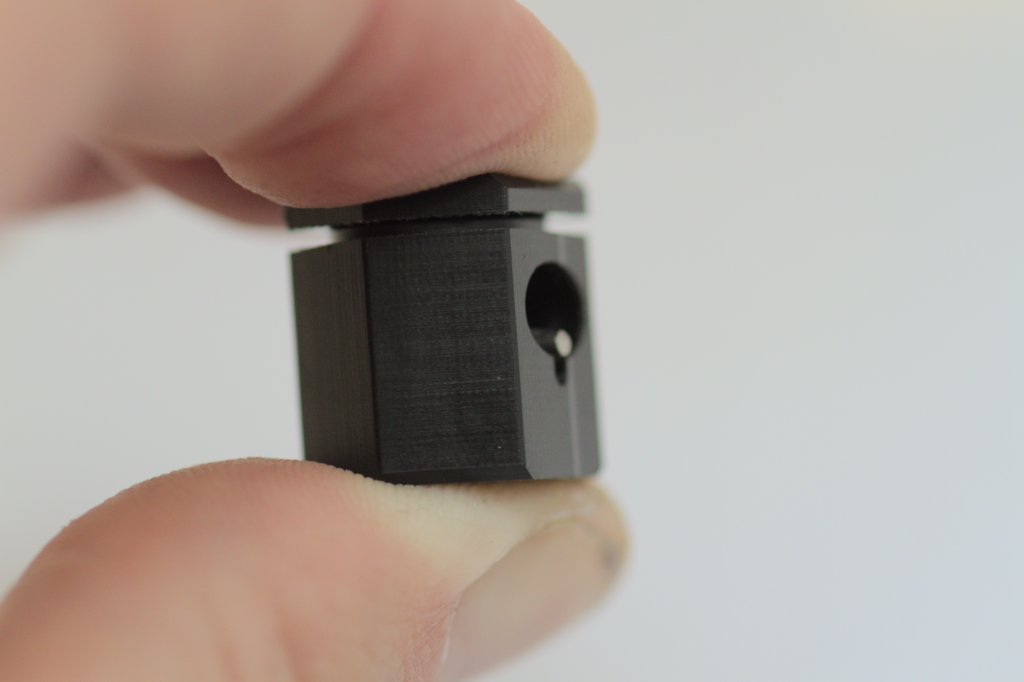
There are some things about the result which were unsatisfactory, which I’ve tried not to emphasize in these photos. They are all addressible with small manufacturing changes, so next versions will be better.